Plants produce many short-interfering RNAs (siRNAs) that are often generated from endogenous loci and repeats or by exogenous agents such as virus. These siRNAs are derived from aberrant double-stranded RNAs and require the activity of RNA-dependent RNA polymerases (RDRs). Biogenesis of siRNAs requires the functions of DCL2, DCL3, DCL4, RDR2, RDR6, and RNA polymerase IV. In some cases, endogenous siRNAs trigger epigenetic effects on target loci and are associated with RNA-directed DNA methylation (RdDM) and chromatin remodeling. RDR6 plays a role in silencing of transgenes, some viruses, and specific endogenous mRNAs that are targets of trans-acting siRNAs (ta-siRNAs). ta-siRNAs are formed by miRNA-guided cleavage within pre-ta-siRNA transcripts (phasing) and through DICER-LIKE processing of dsRNA. The phased ta-siRNAs direct the degradation of target mRNAs as do plant miRNAs. It is predicted that divergence of siRNAs and their targets between species mediate hybrid incompatibility in allopolyploids.
MicroRNAs (miRNAs) are 20-24 nucleotide RNA molecules that play essential roles in posttranscriptional regulation of target genes. tasiRNAs are formed by miRNA-guided cleavage products within primary tasiRNA transcripts, and the phased tasiRNAs direct the degradation or repression of target mRNAs as do plant miRNAs. Unlike siRNAs, many miRNA and tasiRNA loci are conserved in sequences, and unique miRNAs and tasiRNAs were commonly identified in the allotetraploids and their progenitors. In plants, miRNAs predominately mediate degradation of target mRNAs via perfect or near-perfect complementary sequences. MicroRNA targets include a large number of transcription factors, suggesting a role of miRNAs in the control of regulatory networks and cellular growth and development.
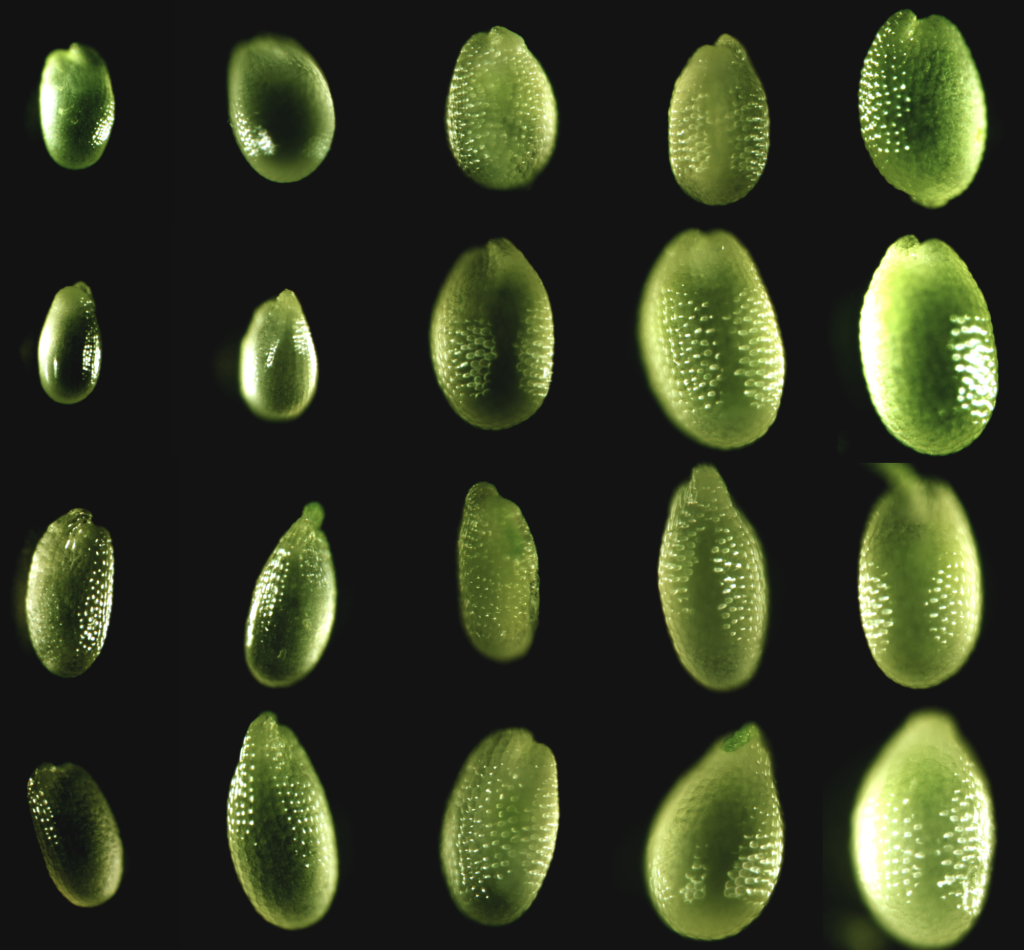
Seed size is important to crop domestication and natural selection and is affected by the balance of maternal and paternal genomes in endosperm. Endosperm, like placenta in mammals, provides reserves to the developing embryo. Interploidy crosses disrupt the genome balance in endosperm and alter seed size. Specifically, paternal-excess crosses (2 × 4) delay endosperm cellularization (EC) and produce larger seeds, whereas maternal-excess crosses (4 × 2) promote precocious EC and produce smaller seeds. The mechanisms for responding to the parental genome dosage imbalance and for gene expression changes in endosperm are unknown. In plants, RNA polymerase IV (PolIV or p4) encoded by NRPD1a is required for biogenesis of a major class of 24-nt small interfering RNAs (also known as p4-siRNAs), which are predominately expressed in developing endosperm. Here we show that p4-siRNA accumulation depends on the maternal genome dosage, and maternal p4-siRNAs target transposable elements (TEs) and TE-associated genes (TAGs) in seeds. The p4-siRNAs correlate negatively with expression levels of AGAMOUS-LIKE (AGL) genes in endosperm of interploidy crosses. Moreover, disruption of maternal NRPD1a expression is associated with p4-siRNA reduction and AGL up-regulation in endosperm of reciprocal crosses. This is unique genetic evidence for maternal siRNAs in response to parental genome imbalance and in control of transposons and gene expression during endosperm development.

We discovered that maternally transmitted siRNAs regulate imprinting, gene expression, and seed size, which may also regulate hybrid incompatibility. Endosperm in cereal seeds like rice and wheat can occupy ~80% of the seed mass, and they provide most calories consumed by humans. Small interfering RNAs (siRNAs) derived from RNA polymerase IV (p4) play a crucial role in regulating gene expression in the endosperm, which resembles the placenta in mammals. We found that a group of p4-siRNAs depends on maternal genome dosage and regulates expression of the target genes such as AGL40 and AGL91, which are involved in endosperm development and seed size. We used laser-capture microdissection (LCM) to separate seed regions and applied AGO4:GFP::AGO4 (promoter:GFP::protein) reporter and promoter:GUS sensors to monitor spatial-temporal patterns of siRNA-mediated silencing. Use of AGO4:GFP::AGO4 promoter sensors has illuminated how maternal siRNAs control the timing and location of gene expression in the seed, impacting overall seed size. These new findings raise new questions to test whether siRNAs move between different seed compartments (endosperm, embryo, seed coat) and how this movement affects seed development, as well as to explore whether maternal siRNAs can regulate paternal genes, potentially contributing to hybrid incompatibility or weaknesses in hybrids.
Moreover, we applied single-cell RNA analysis to analyze correlations between transcript abundance, cell size and ploidy levels. An increased activity of DEMETER in the tetraploid central cell compared to the diploid may result in de-repression of polycomb-group repressive complex2 (PRC2) genes. In addition, abundant transcription of the genes encoding for cysteine-rich peptides in central, synergid, and egg cells may indicate their roles in cell-cell recognition during double fertilization. These findings provide a foundation for addressing key questions about the roles of maternal and paternal contributions to seed development in hybrid plants.
Hybrid Incompatibility

Although heterosis is common in hybrids and allopolyploids that overcome postzygotic barriers, some hybrids and allopolyploids are sterile and cannot produce offspring, a phenomenon known as hybrid incompatibility. Dobzhansky (1936), Muller (1942), and Bateson (1909) proposed a model to explain the phenomenon. The modern iteration of their model is that hybrid incompatibilities are caused by interactions between genes and small RNAs that have functionally diverged in the respective hybridizing species. In Drosophila, piRNAs are associated with the Argonaute proteins Piwi and MiWi2, and loss of protein function and/or maternal transmission of siRNA in the repression of transposition cause sterility or hybrid dysgenesis. In Arabidopsis, genome-wide demethylation and maternal transmission of siRNAs were observed in endosperm. In allotetraploids we found accumulation of Arabidopsis thaliana (maternal) centromeric siRNAs associated with DNA methylation. Moreover, many siRNAs are absent in F1 but are restored in later generations, suggesting that these siRNAs affect genome stability through interactions between siRNAs and centromeric proteins. One possibility is that siRNAs produced in one species cannot be recognized by proteins, including histone variants, centromeric and/or siRNA biogenesis proteins, in another species, failing to silence heterochromatin and transposons, leading to “genome shock” as predicted by Barbara McClintock (1984) and seed lethality. Using Arabidopsis interspecific hybrids and genetically tractable allopolyploids, we will test how hybrid lethality occurs during interspecific hybridization and how they are overcome during self-pollination to produce fertile and stable allopolyploids.
Five Selected Publications
- Lu, J., Zhang, C., Baulcombe, D. C., and Chen, Z. J. (2012) Maternal siRNAs as regulators of parental genome imbalance and gene expression in endosperm of Arabidopsis seeds. Proc. Natl. Acad. Sci. USA 109:5529-5534.
- Kirkbride, R. C., Yu, H. H., Nah, G., Zhang, C., Shi, X., Chen, Z. J. (2015) An epigenetic role for disrupted paternal gene expression in postzygotic seed abortion in Arabidopsis interspecific hybrids. Molecular Plant 8:1766-1775.
- Kirkbride, R. C., Lu, J., Zhang, C., C., Mosher, R. A., Baulcombe, D. C., Chen, Z. J. (2019) Maternal small RNAs mediate spatial and temporal regulation of gene expression and seed development in Arabidopsis. Proc. Natl. Acad. Sci. USA 116:2761-2766.
- Song, Q., Ando, A., Jiang, N., Ikeda, Y., Chen, Z. J. (2020) Single-cell analysis reveals ploidy-dependent and cell-specific transcriptome changes in Arabidopsis female gametophytes. Genome Biol. 21:178.
- Cao, S., Wang, L., Han, T., Ye, W., Liu, Y., Sun, Y., Moose, S. P., Song, Q., Chen, Z.(2022)Small RNAs mediate transgenerational inheritance of genome-wide trans-acting epialleles in maize. Genome Biology 23:53.