In the past month or so, we have seen time and time again studies and news stations reporting that immunity to SARS-CoV-2 begins to wane within a few weeks of infection and is largely absent by 3 months post-infection. As scary as this sounds, it does not necessarily mean that there is no hope for establishing herd immunity or ever doing away with COVID-19. No immunity is everlasting – even the longest-lived memory B cells will die after 40 years or so – and even viruses for which we have highly successful vaccines require multiple rounds of vaccination to build up a successful immune response and create memory (this is due in part to the modality of the vaccines being used: attenuated virus vaccines are a popular choice because they are relatively easy to make and have little or no chance of reverting back to a pathogenic strain, but because they are not fully virulent, they do not induce a full immune response and thus require boosters). Memory is the key. When people speak of “waning immunity”, what they mean is that levels of the potent IgG and IgM antibodies that circulate and recognize the specific pathogen begin to drop after a few weeks, but this is expected, given that the half-life of IgG is 10-21 days across the board, for almost every known pathogen (Booth et al., 2018). Figure 1 highlights the general immune response to SARS-CoV-2 infection and outlines a predicted time course for the adaptive immune response.
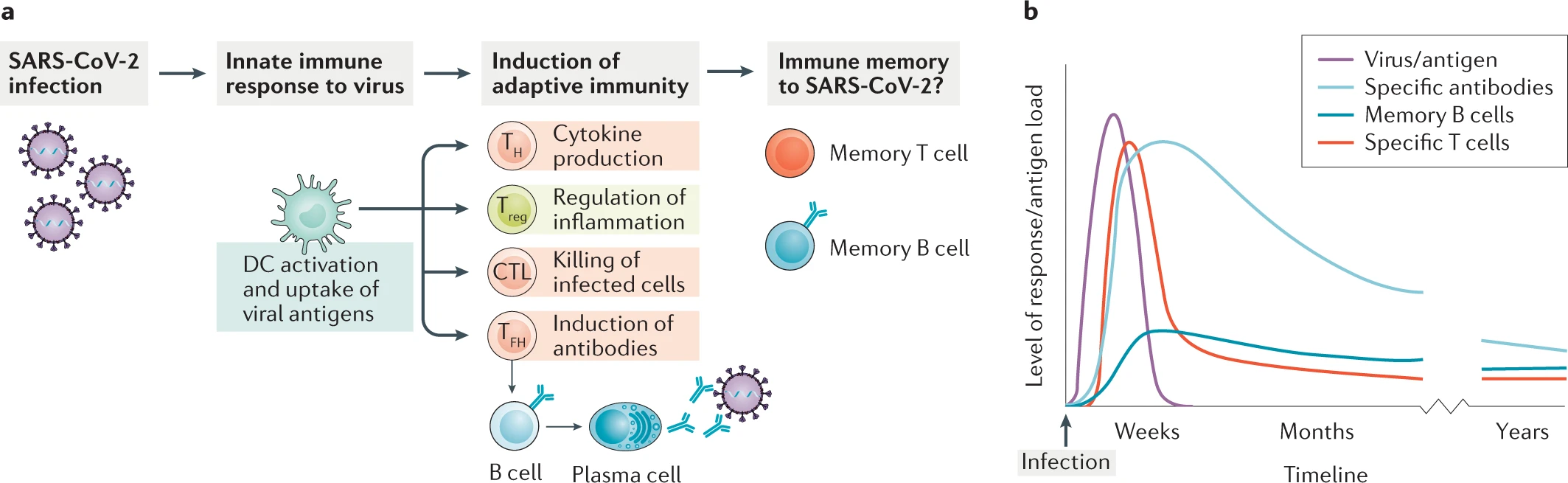
In SARS-CoV-2 infection, as in most viral infections, the antibody response produced by plasmablasts (one group of terminally differentiated B cells) depends on both the viral load – i.e., the amount of virus with which one is infected – and duration of active infection. Once all actively replicating virus has been removed from the body, the immune system stops receiving the stimulation necessary for the differentiation of B cells and production of antibodies. Antibodies specific for the spike protein of SARS-CoV-2 have been found in patients with disease severity ranging from mild to severe, and have even been found in asymptomatic individuals, suggesting some amount of cross-reactivity with spike proteins of more widely-circulating human coronaviruses (Long et al., 2020). What’s more, T cells against the SARS coronavirus have been found to cross-react with SARS-CoV-2, almost 17 years later (Bert et al., 2020). Additionally, immune responses consisting of spike protein-specific neutralizing antibodies, and memory B and T cells have been recorded in convalescent COVID-19 patients (Juno et al., 2020). And SARS-CoV-2-specific CD4+ and CD8+ T cells have been found in patients recovered from COVID-19 that were shown to recognize not only the spike protein, but also peptides from the nucleocapsid and matrix proteins, in addition to several others (Grifoni et al., 2020). This bodes well, not only for the maintenance of a long-term immune response to SARS-CoV-2, but also for the development of a vaccine that will offer protection far into the future.
These data all point to the intricate and multi-modal nature of the immune response – our immune systems are not one-trick ponies that rally the same antibody response for every infection. Instead, they boast complex regulated pathways that employ both immediate fixes (in the form of antibodies) and far-reaching solutions (immune memory B and, especially, T cells). Despite the talk of our bodies’ inability to offer permanent protection against SARS-CoV-2, it is important to keep in mind that the immune system is more than just antibodies; the development of immune memory is ultimately what will enable herd immunity and keep us safe from infection in the future.
References
Bert, N. L., Tan, A. T., Kunasegaran, K., Tham, C. Y. L., Hafezi, M., Chia, A., Hui, M., Cheng, Y., … Tan, Y. J., & Bertoletti, A. (2020). SARS-CoV-2-specific T cell immunity in cases of COVID-19 and SARS, and uninfected controls. Nature, 584, 457-462. https://doi.org/10.1038/s41586-020-2550-z
Booth, B. J., Ramakrishnan, B., Narayan, K., Wollacott, A. M., Babcock, G. J., Shriver, Z., & Viswanathan, K. (2018). Extending human IgG half-life using structure-guided design. MAbs, 10(7), 1098–1110. https://doi.org/10.1080/19420862.2018.1490119
Cox, R. J., Brokstad, K. A. (2020). Not just antibodies: B cells and T cells mediate immunity to COVID-19. Nat Rev Immunology, 20, 581-582. https://doi.org/10.1038/s41577-020-00436-4
Jiskoot, W., Kersten, G. F. A., Mastrobattista, E., & Slütter, B. (2019). Vaccines. Pharmaceutical Biotechnology: Fundamentals and Applications (pp. 282–304). Springer International Publishing. https://doi.org/10.1007/978-3-030-00710-2_14
Juno, J. A., Tan, H. X., Lee, W. S., Reynaldi, A., Kelly, H. G., Wragg, K., Esterbauer, R., Kent, H. E., … Kent, S. J., & Wheatley, A. K. (2020). Humoral and circulating follicular helper T cell responses in recovered patients with COVID-19. Nature Medicine, 26, 1428-1434. https://doi.org/10.1038/s41591-020-0995-0
Long, Q. X., Tang, X. J., Shi, Q. L., Li, Q., Deng, H. J., Yuan, J., Hu, J. L., Xu, W., Zhang, Y., Lv, F. J., … Chen J., & Huang A., L. (2020). Clinical and immunological assessment of asymptomatic SARS-CoV-2 infections. Nature Medicine, 26, 1200-1204. https://doi.org/10.1038/s41591-020-0965-6