Background:
Global warming poses the most important challenge to mankind. In addition to exploring renewable energy resources, recycling and utilization of greenhouse gases to synthesize useful products is also necessary to the mitigate climate problem. The two most important greenhouse gases (GHG) are methane (CH4) and CO2, and methane has >20 times the GHG potential than CO2 [IPCC, 2014]. Methane is the main content of natural gas, which usually coexists with petroleum. When the oil extraction facilities locate in areas without natural gas transport capability (pipes or liquidation equipment), natural gas can cause explosion during oil extraction by inducing extremely high and unstable pressure. To eliminate this risk, gas flaring (burning of natural gas) has been a common practice for over 160 years. In 2021, flared nature gas releases over 400M tons of CO2 worldwide. A similar situation applies to landfill gas (LFG), which contains roughly 50% CH4 and 50% CO2. In 2020, 14.5% of the US methane emission came from LFG, the third largest in the category [epa.org].
To address these problems, developing small-scale, flexible and economically feasible gas utilization technologies has great potential. Current techniques, like small power plants for electricity generation and liquefied natural gas plants mounted on trucks [afdc.energy.gov], are often expensive and not economically practical. An alternative solution is to convert CH4 to other products with high energy density, such as ammonia (NH3) and liquid H2, both of which are considered as carbon-free green fuels. Traditionally NH3 has been used mainly as a nitrogen fixed compound for fertilizer production. Compared to liquid H2, converting CH4 to NH3 is advantageous because of its higher energy density (Fig. 1c) and low storage and transportation cost.
Fig. 1. (a) Flared natural gas; (b) 2020 U.S. methane emissions; (c) Energy density of various energy resources.
Non-thermal Plasma
NH3 is typically produced in large-scale facilities, via methane steam reforming and the Haber-Bosch processes that require high temperature (around 500°C) and high pressure (around 150 bar) environment. Non-thermal plasma, which contains highly-active charged ions and electrons, can enable and accelerate chemical reactions in an efficient manner. Non-thermal plasmas are generated through ionization of chemical species in high electromagnetic field. Electron temperature in non-thermal plasma can be higher than 10,000K, while the gas temperature is much lower (< 500K typically). Non-thermal plasma technology has great advantages including high energy density, low power input, fast start time, environmentally safe, scalable, flexible and compatible with renewable source of energy, such as wind and solar.
Non-thermal plasma can be generated with microwave, direct-current, and radio-frequency excitation, among others [Ong et al., 2022; George et al., 2021; Liu et al., 2020]. Of these, the microwave plasma is promising for operation at atmospheric pressure because of its high ionization efficiency, low electron temperature with corresponding low floating potentials that are less damaging to surfaces bounding the plasma.
Our Approach:
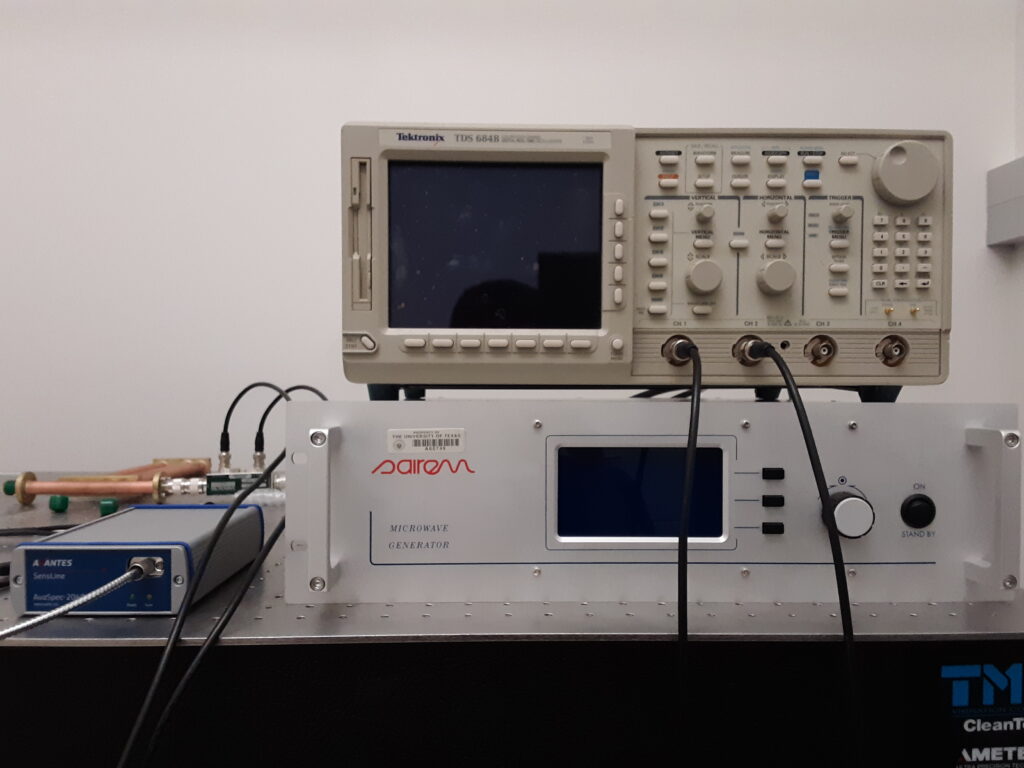
Figure 2. Equipment for generating microwaves and for measurement of the non-thermal plasma. Pictured here are a Sairem GMS-200 microwave source, an oscilloscope for measurement of forward and reflected power, and a spectrometer which is used measure properties of the plasma.
Our focus is to develop a small-scale, simple, low-cost and highly flexible microwave plasma device to convert methane to ammonia with high efficiency. Our approaches include:
(i) Multi-physics modeling of non-thermal plasma generation, propagation and integration with chemical flow. (Collaborate with plasma theorists)
(ii) Understand the fundamental knowledge about plasma-flow/catalyst interactions with optical spectroscopic measurements
(iii) Design, fabricate and optimize the device for commercial usage.